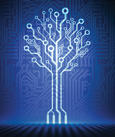
Spanning Tree Protocol
How the Spanning Tree protocol organizes an Ethernet network
Organizations today often divide their local networks using switches, which operate Layer 2 of the OSI model, rather than the (Layer 3) routers for which the TCP/IP network protocol system was originally designed. Switches are inexpensive and quite effective for reducing traffic and subdividing the network, but, in fact, a switch is still operating at the Ethernet level and doesn't have access to the time-to-live (TTL) settings, logical addressing, and sophisticated routing protocols used with routers.
Networks that are subdivided with multiple switches could theoretically experience a situation where an Ethernet frame loops continuously around the network, forwarded endlessly through the circle of switches – but they don't. One of the main reasons why they don't is the Spanning Tree protocol, which was specifically designed to address this problem of Ethernet loops and has been adopted (and adapted) by many of the biggest switch vendors.
Spanning Tree prevents network loops from occurring and causing broadcast storms that would very quickly overload a network segment. The Spanning Tree protocol performs this magic by disabling certain connections between switches. Modern switches use a spanning tree to determine routes through the switched network – and to close off routes that could potentially cause a loop. The devices are designed to map these routes automatically, but if you need to do some troubleshooting or address performance issues on your Ethernet network, it helps to have some basic knowledge of Spanning Tree.
Understanding Spanning Tree
Figure 1 shows three examples (A, B, C) of topologies that would lead to the collapse of a network without a spanning tree blocking some switch ports.
...Buy this article as PDF
(incl. VAT)